Motor vibration analysis
Electric motors are used in a wide range of industrial applications. In the United States alone, there are over 1.2 billion electric motors of all types. Motors account for 78% of the electrical energy consumed in industrial systems, rising to 90% for process industries. [1]
Many of these motors are critical to safety or efficient operations, making their reliability of vital importance. Monitoring and analysing motor or machine vibrations can supply data for predictive maintenance programmes, allowing engineers to identify and repair problems before they cause reduced performance or failures.
A growing number of industries are becoming aware of the importance of motor vibration analysis. Motor applications that can benefit from machine vibration analysis include rotating equipment such as pumps and fans, gear boxes, compressors, bearings, and wind turbine generators.
Some industries are particularly reliant on rotating machinery. The food and beverage industry for example, uses many compressors, chillers and pumps during processing. Monitoring these for motor vibrations allows timely intervention to prevent downtime that could lead to ingredients or production batches being spoiled.
The water industry needs to avoid downtime in its pumps to maintain water quality and supply pressures to customers. Machine vibration analysis helps keep the pumps healthy and allows the utility to avoid the risks of fines and sanctions for non-supply.
What causes motor vibrations?
While it is normal for motors to generate small vibrations, large vibrations or any changes in motor vibration can indicate problems. The total vibration experienced by a motor can be down to many elements and knowing what these possible causes can be allows a better diagnosis of what is happening to the motor.
One of the prime causes of vibration is an imbalance: a spot where an unbalanced weight moves around the machine’s axis, causing the rotating components to vibrate. Imbalances can be caused by casting flaws or machining errors or maintenance issues such as dirty fan blades.
Bearings can be a major cause of vibrations. Loose bearings can lead to vibration spreading to other components. A lack of lubrication makes bearing components wear out quickly. A rutted roller bearing race can create movement each time a bearing travels over the damaged area.
Gears are also a potential source of vibration if they become misaligned. Worn or broken gear teeth can grind against each other, causing a potentially hazardous vibration.
Another factor in vibrations is the stability of the mounting that the motor is attached to. For example, if a motor is loosely attached to its mounting, vibration can cause more damage to the bearings. Incorrect mounting can also cause wear and fatigue among the mounts and other motor components, such as the motor’s insulation.
Inadequate mounting can also cause the machine’s shafts to become misaligned. This can take the form of angular misalignments, when the axes and pump on a motor are not parallel, or parallel misalignment when the axes are parallel but are misaligned. This can be caused by improper assembly of the motor and its drive chain or can develop as a result of use over time – the latter can result from faulty reassembly, thermal expansion or components shifting their position.Vibration can also result from external sources, such as machines or other processes that connect to the motor.
The impact of motor vibrations
Vibrations could indicate problems that reduce the energy efficiency of the motor, increasing cost. Ensuring that motor components are aligned and not suffering undue wear can help boost the energy efficiency of motors and thus cut operating costs. Although this is important, particularly in a climate of increasing energy prices, the biggest problems arise when a motor fails. This can potentially cause unplanned downtime in a factory, where there can be significant losses incurred while the motor is repaired. For this reason, a survey of motor users found that more than 70% cite reliability as the first priority of their motor maintenance programmes.
Failure costs can vary across industries, but many factories will have bottleneck processes where the failure of one motor can have a significant impact on the profitability of the plant. Figures produced by ABB Motors outlined the potential costs arising if a motor fails.
The analysis took the case of a 315kW motor with a 95.5% efficiency used in a continuous process. At an energy cost of 11p/kWh, and with the motor running for 8,400 hours per year, the cost of running the motor over a 20-year lifetime would be £6,094,704. This is extremely high compared to the typical purchase cost of £18,000.
Yet the cost of not running the motor is equally significant. The analysis cited the example of a motor used in the oil and gas industry, a failure of which could lead to losses of £220,000 an hour. Just one ten hour failure over the motor’s 20 year life span would thus lead to losses of £2,200,000. Similarly large losses are also possible in industries such as automotive, metal casting and food and beverage.
Although some equipment faults are instantaneous, most catastrophic faults that affect production are the results of poorly implemented or even non-existent, maintenance programmes. These failures are largely due to the management viewing maintenance as an expense to be minimised if possible, rather than its rightful status as an investment in the future of the business.
Motor vibration monitoring
A number of different sensors can be used to measure motor vibration, each working on slightly different physical principles to detect movements in the motor under test. Some sensors work through direct contact methods and so require mounting onto the motor casing, while non-contact devices can detect movement at a distance through optical or magnetic means.
Accelerometers are the most commonly used method and work by measuring the change in velocity of the component. These manifest as vibrations that cause the accelerometer to produce an electrical signal that can be processed to produce useable vibration data. Piezoelectric accelerometers are the most widely used type, as they produce a strong, clear signal at most frequencies.
Other commonly used types of sensor are strain gauges and microphone sensors. Strain gauges detect vibrations by measuring the time taken for an electric current to pass through a grid that deforms in response to movement. Microphone sensors can detect changes in high frequency sounds and are a cost-effective method of providing some basic information.
Another possibility is to employ handheld vibration meters that can be used to give a rapid indication of vibration signals, and thus indicate that a particular machine may be suffering from a fault. Many maintenance technicians might use a vibration meter along with a sound level meter to analyze frequencies. These meters typically have a memory for storing measurements and often a data logger for collecting and storing data over longer periods.
A typical vibration meter is the Wilcoxon MAC800 series. This device offers built-in band filters for detecting faults such as unbalance, misalignment or looseness. Its low frequency range allows readings to be taken on machinery running as slowly as 120 rpm.
Although they are useful in allowing maintenance managers to measure vibrations in motors and other equipment without fixed sensors, the latter are generally better as they monitor continuously.
Motor vibration analysis
Successful motor vibration analysis involves quantifying the captured vibration signal into several metrics, then tracking and comparing those metrics over time. There are three broad categories of analysis: time-domain, frequency-domain and artificial intelligence algorithms.
- Time domain analysis
- Frequency domain analysis
- AI in condition monitoring for motors
Some of the more commonly used metrics are used in time domain analysis. These are peak acceleration, RMS acceleration, crest factor, velocity RMS and displacement RMS.
Peak acceleration is easy to see and calculate. It plots the amplitude of the vibration from the highest point of the recorded waveform to the lowest point and plots this against the time axis. This gives a figure for acceleration in Gs. Peak acceleration values can be too dependent on the sampling rate of the signal. This means that when comparing multiple signals, different sample rates can make comparison between the peak accelerations inappropriate.
RMS acceleration is preferred to peak acceleration because it is independent of the sample rate and so gives a more accurate comparison of the vibration level of two signals. RMS is also more likely to correlate with the vibration energy. Overall, acceleration RMS is a popular and useful metric for vibration health monitoring. Crest factor is the ratio of the peak acceleration to the RMS acceleration – as such, it defines how ‘peaky’ a signal is. For example, a square wave would have a crest factor of 1, while a signal containing intermittent shock events could have a very high crest factor. It is useful because an increasing crest factor over time tends to indicate bearing failure.
An acceleration signal can be integrated to get the velocity (proportional to energy) and displacement. The equivalent RMS values can be calculated to give velocity RMS and displacement RMS. Velocity RMS is an important metric as there is an ISO standard, 10816, that defines the vibration severity for different classes of machines. Because velocity corresponds to energy, it is the main metric tracked over time to monitor vibration health and inform predictive maintenance programmes.
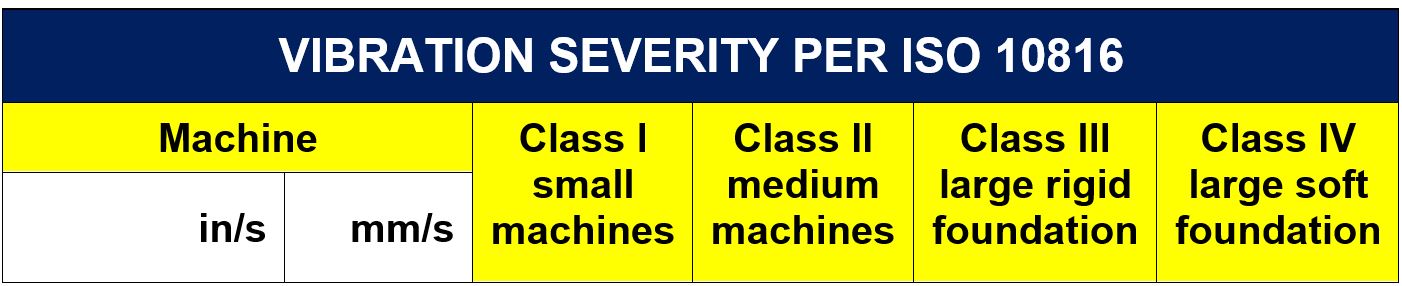
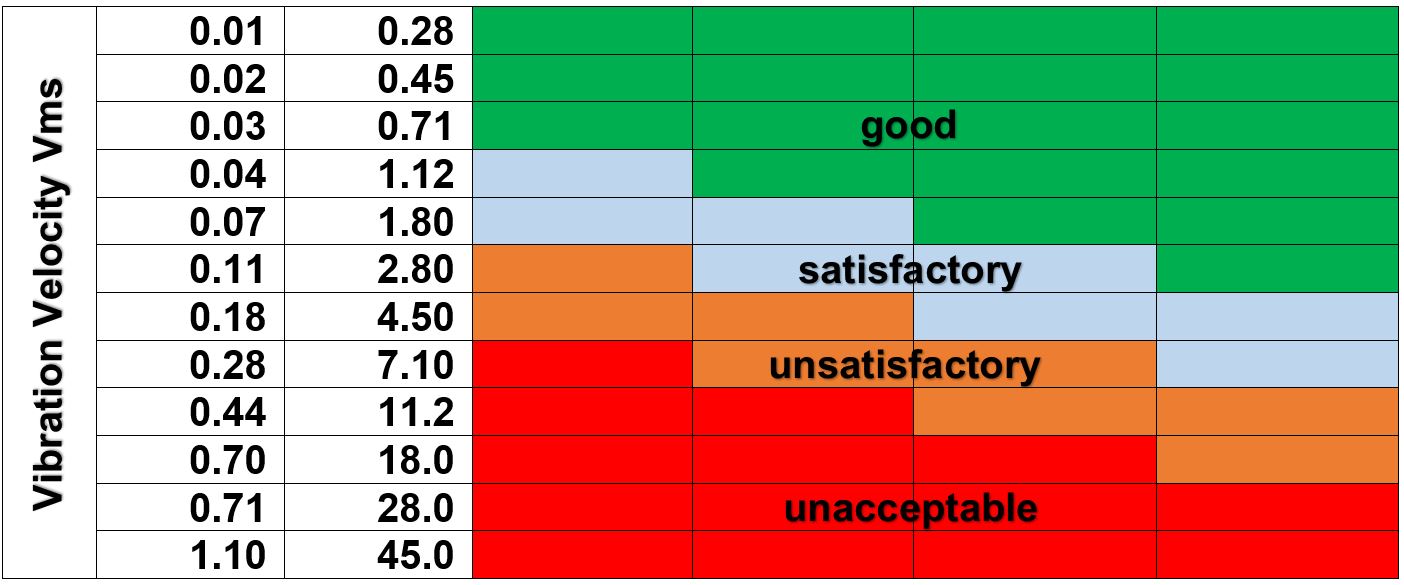
Figure 1: Table of vibration severity as defined by ISO 10816
Displacement RMS is not as important as velocity RMS, as displacements tend to occur only at very low frequencies, meaning the displacement values will often be very low. However, they are useful in rotating equipment, as unbalanced shafts and other components can cause significant displacements.
The vibrations experienced by a machine are composed of different frequencies. For example, the total vibration may be made up of many different vibrations from the motor, the bearing, the drive shaft or numerous other components. To analyze these many frequencies, Fast Fourier Transforms (FFT) are used. The technique decomposes the signal into all its constituent frequencies, converting it from the time domain into the frequency domain.
This process gives acceleration/vibration amplitude as a function of frequency. This allows investigators to perform analysis in the frequency domain to give a deeper understanding of the vibration profile. Most vibration analysis will be done in the frequency domain.
FFTs are best at analyzing vibration in which there are a limited number of dominant frequency components. When characterizing random vibration signals, Power Spectral Densities (PSD) are used instead. This allows comparison of vibration levels in signals that have different lengths.
One of the major tools in predictive maintenance is Artificial Intelligence (AI). Combined with Machine Learning (ML), its offers great scope to improve the availability of motors while cutting costs. According to McKinsey & Company, AI-based predictive maintenance can improve availability by up to 20%, cut inspection costs by 25% and reduce annual maintenance fees by up to 10%.
ML works by using algorithms to operate on a set of training data relating to factors such as asset condition and performance, failure rates, maintenance processes, load and duty cycles and environmental conditions. Using this data, the algorithm creates a mathematical model to describe the complex system and the interactions of its components. The goal is to predict a dependent “target” variable such as asset health or remaining lifetime. When fresh real world data is put into the model, based on actual measurements, the model will return a status or prediction for the motor, allowing engineers to make informed decisions about its maintenance.
Much of the work involved in AI based methods can be avoided by using cloud based solutions such as Senseye. Using data already collected, the solution generates behaviour models to help engineers pay attention to critical issues.
Some vibration sensors can easily integrate with AI software to build a condition monitoring system. An example is the STM32 sensor board from ST, which allows the user to set up vibration based condition monitoring solutions that uses anomaly detection AI libraries.
Predictive and preventive maintenance
The aim of measuring vibrations is to ensure that motors perform optimally, delivering the required performance and their designed energy efficiency for as long as possible. Motor operators need to ensure high availability of their motors, particularly for critical processes that cannot be interrupted without causing significant losses.
Although preventive maintenance remains popular, this is dictated by pre-set maintenance intervals that pay little attention to the actual health of the machine. Because they don’t necessarily align with production schedules, they can be disruptive and thus lead to losses.
By contrast, predictive maintenance assesses the condition of equipment by performing intermittent or continuous condition monitoring
Motor vibration monitoring plays a crucial role here – by ensuring that motor users always have an up-to-date assessment of their motors’ condition, they can ensure they receive the maintenance they need at the correct time, maximizing availability, maintaining efficiency and cutting costs.
Stay informed
Keep up to date on the latest information and exclusive offers!
Subscribe now
Thanks for subscribing
Well done! You are now part of an elite group who receive the latest info on products, technologies and applications straight to your inbox.